Mitochondrial genome anatomy and species-specific lifespan
- Gilad Lehmann
- 31 ביולי 2017
- זמן קריאה 8 דקות
The reasons for some animals being long-lived and others short-lived, and, in a word, causes of the length and brevity of life call for investigation.
— Aristotle, On Longevity and Shortness of Life (350 B.C.)
Humans and animals have two different DNAs: (1) nuclear DNA and (2) mitochondrial DNA (mtDNA). The nuclear DNA resides is in the cell nucleus and the mtDNA resides in the cell mitochondria. The consequence of the different localization is that the metabolic environment as well as the repertoire of proteins is different. This includes the DNA repair and maintenance machineries.
Over the last decade, a particular phenomenon was observed which suggests that the mtDNA is the important determinant of animals maximum lifespan (MLS). This evidence is that the chemical composition of the mtDNA (and not the nuclear DNA) correlates with animals characteristic maximum lifespan (MLS; figure 1) [1,2,3,4]. The mtDNA chemical composition is actually its base composition (A, T, G, and C) and therefore it is quantitatively measurable. Figure 1 presents the correlation between the mtDNA GC-content and maximum life span across 387 different species of mammals.
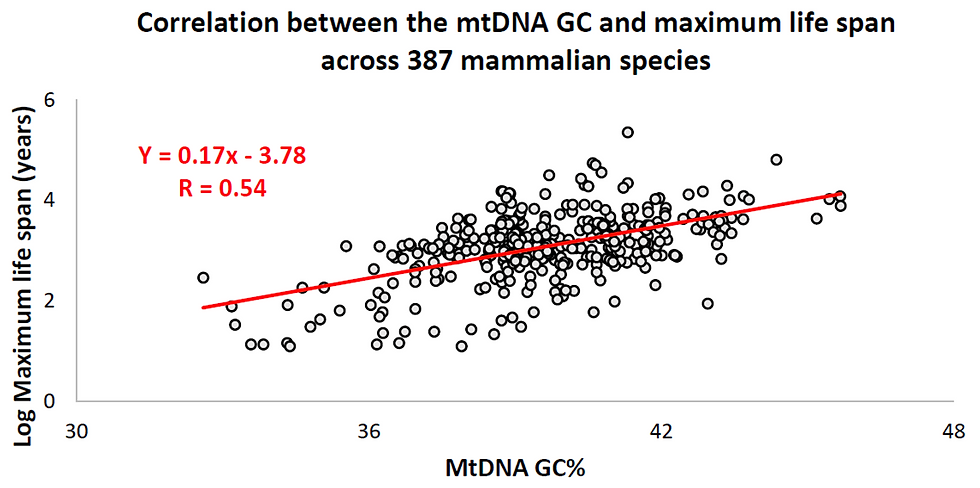
Figure 1. Unbelievable correlation between the mtDNA GC% and mammalian species lifespan.
The correlation between the mtDNA GC and MLS (Figure 1) is considered strong in the field of biology where extremely complex systems are studied. Further strengthening the confidence in the link between the mtDNA and MLS is that another feature of the mtDNA correlates with MLS - its size [5]. Besides the mtDNA GC, another factor is known to correlate with animals MLS - resting metabolic rate (RMR)[6,7,8,9,10,11]. RMR is an approximation to the minimal energy expenditure of animals and is measured at a condition of rest. It therefore represents the low threshold of energy usage just to stay alive. The RMR correlates tightly with MLS but it does not correlate with the mtDNA base composition [2]. Furtheremore, the RMR and the mtDNA base composition explain different parts of the variability in animals MLS [2,3,5]. As follows, the mtDNA and RMR can be used as two independent predictors of MLS (from statistical perspective). The practical implementation of this notion is illustrated in figure 2 where the combination of mtDNA and RMR explains 3/4 of the MLS differences among mammals. In other words, the combination of mtDNA and RMR allows to generate the strongest correlations with MLS that have been reported to date (for comparable sample size of animals, i.e., not just 3 species). The mathematical function for the dependence of MLS on mtDNA and the RMR is multiplicative (MLS = e^mtDNA * RMR, or; logMLS = mtDNA + logRMR) and suggests that although the mtDNA and RMR are independent (statistically), they do interact in determination of MLS.

Figure 2. Combined, the mtDNA GC% and RMR explains ~75% of the variation in mammals longevity.
It is a matter for debate why the mtDNA base composition and RMR correlate with MLS, and why they seem to be statistically independent from each other. A possible explanation is that the mtDNA represents the pathway for energy production by the mitochondria while the RMR represents the cellular pathways for energy comsumption. The energy production and energy consumption pathways are independent from each other but could interact in determination of MLS. Thus, the mtDNA GC and RMR are two sides of a same coin. On one side of the coin, the mtDNA base composition reflects a capability to protect the mtDNA and preserve the ability to generate energy from food and on the other side, the RMR represents an ability to contain a decrease in the mitochondrial energy generation. For illustration, a mouse with high RMR is susceptible to decline in its mitochondrial ability to generate energy while turtles and elephants with very low RMR are can endure such decline for longer time (and thus live longer).
Alternative explanations for the mtDNA and RMR correlation with MLS are that they are both mediated by radical oxygen species (ROS). Higher metabolic rate is associated with higher ROS production rate, and changes in the mtDNA base composition are associate with change in the mitochondrial proteins that produce ROS, presumably leading to less ROS production by the mitochondria [12,13,14,15,16]. Another alternative is that the amino acids changes alter the stability and vulnerability of the mtDNA coded proteins which are important for longevity [16,17]. Specifically, it has been suggested that accumulation of threonines and serines in the mtDNA encoded proteins is advantegous for longevity [18,19], that less cystein is advantageous for longevity [20], and that less methionine is advantageous [21]
It is not clear how to successfuly translate the findings on the mtDNA-MLS links into action items. The SENS Research Foundation has set as a goal to make backup copies of the mtDNA genes in the nuclear DNA (a process called allotopic expression). Copies of mtDNA chunks already exist in the nuclear DNA and they are called NUMTs (for nuclear mitochondrial segment). As far as we know, these NUMTs are not functional because the mtDNA and nuclear DNA have different genetic codes, including different stop codons. In one lizard however, that is named Sphenodon punctatus (tuatara), the mtDNA lacks the genes ND5, tRNA(His) and tRNA(Thr) which are suggested to have moved to the nucleus [22]. According to the records in the AnAge database, the tuatara lizard weights less than 1 kg and can live 90 years and probably more [23]. This supports the potential direction of lifespan prolongation by allotopic expression. Another potential action item could involved the study of mitochondrial transfer between cells [24], a process that can rescue cells with defective aerobic respiration [25,26,27,28,29]. A yet different possibility is to study the ability of young people stem cells to rejuvenate old people. More possibilites exist.
To accelerate the research on the link between the mtDNA and MLS and to demonstrate the reliability the data, the MitoAge database was constructed [30]. MitoAge contains calculated mtDNA compositional features of the entire mitochondrial genome, mtDNA coding (tRNA, rRNA, protein-coding genes) and non-coding (D-loop, insertions) regions, codon usage for each protein-coding gene, and longevity records for over 900 species from all taxa of the Kingdom Animalia. By downloading the data from MitoAge, anyone can in short time reproduce figure 1 and beyound, and observe the correlative links between the mtDNA and MLS.
Below are presentations that I gave about this research in two conferences.
Presentation 1. Mitochondrial determinants of mammalian longevity. Understanding Aging: Biomedical and Bioengineering Approaches, UCLA, Los Angeles, CA, June 28-29, 2008.
Presentation 2. Mitochondrial Determinants of the Mammalian Life Span. Eurosymposium on Healthy Ageing. A new age of long term health and longevity. Brussels, December 12-14, 2012.
References
Lehmann, Gilad; Budovsky, Arie; Muradian, K. Muradian; Fraifeld, Vadim E. (2006). "Mitochondrial genome anatomy and species-specific lifespan". Rejuvenation Res. 9 (2): 223–226. PMID 16706648. doi:10.1089/rej.2006.9.223.
Lehmann, Gilad; Segal, Elena; Muradian, K. Muradian; Fraifeld, Vadim E. (2008). "Do mitochondrial DNA and metabolic rate complement each other in determination of the mammalian maximum longevity?". Rejuvenation Res. 11 (2): 409–417. PMID 18442324. doi:10.1089/rej.2008.0676.
Lehmann, Gilad; Muradian, K. Muradian; Fraifeld, Vadim E. (2013). "Telomere length and body temperature-independent determinants of mammalian longevity?". Front Genet. 4 (111). PMID 23781235. doi:10.3389/fgene.2013.00111.
Samuels, David C. (2005). "Life span is related to the free energy of mitochondrial DNA.". Mech Ageing Dev. 126 (10). PMID 15992863. doi:10.1016/j.mad.2005.05.003.
Lehmann, Gilad; Segal, Elena; Tacutu, Robi; Budovsky, Arie; K. Muradian, Khachik; E. Fraifeld, Vadim (2008). "Mitochondrial determinants of mammalian longevity" (PDF). Problems of Aging and Longeivty.
Rubner, M. (1908). Das Problem det Lebensdaur und seiner beziehunger zum Wachstum und Ernarnhung. Munich: Oldenberg.
Raymond Pearl. The Rate of Living. 1928
Harman, D. (July 1956). "Aging: a theory based on free radical and radiation chemistry". Journal of Gerontology. 11 (3): 298–300. ISSN 0022-1422. PMID 13332224.
Harman, D. (April 1972). "The biologic clock: the mitochondria?". Journal of the American Geriatrics Society. 20 (4): 145–147. ISSN 0002-8614. PMID 5016631.
Speakman, John R.; Selman, Colin; McLaren, Jane S.; Harper, E. Jean (June 2002). "Living fast, dying when? The link between aging and energetics". The Journal of Nutrition. 132 (6 Suppl 2): 1583S–97S. ISSN 0022-3166. PMID 12042467.
Speakman, John R. (May 2005). "Body size, energy metabolism and lifespan". The Journal of Experimental Biology. 208 (Pt 9): 1717–1730. ISSN 0022-0949. PMID 15855403. doi:10.1242/jeb.01556.
Rottenberg, Hagai (2006). "Longevity and the evolution of the mitochondrial DNA-coded proteins in mammals.". Mech Ageing Dev. 127 (9): 748–60. PMID 16876233. doi:10.1016/j.mad.2006.06.001.
Rottenberg, Hagai (2007). "Coevolution of exceptional longevity, exceptionally high metabolic rates, and mitochondrial DNA-coded proteins in mammals.". Exp Gerontol. 42 (4): 364–373. PMID 17137741. doi:10.1016/j.exger.2006.10.016.
Rottenberg, Hagai (2007). "Exceptional longevity in songbirds is associated with high rates of evolution of cytochrome b, suggesting selection for reduced generation of free radicals.". J Exp Biol. 210: 2170–2180. PMID 17562891. doi:10.1242/jeb.004861.
Rottenberg, Hagai (2014). "Exceptional longevity and exceptionally high metabolic rates in anthropoid primates are linked to a major modification of the ubiquinone reduction site of cytochrome b.". J Bioenerg Biomembr. 46(5): 435–445. PMID 24827527. doi:10.1007/s10863-014-9552-1.
Mariadassou, Mahendra; Pellay, François-Xavier (August 2014). "Identification of amino acids in mitochondrially encoded proteins that correlate with lifespan". Experimental Gerontology. 56: 53–58. ISSN 1873-6815. PMID 24657631. doi:10.1016/j.exger.2014.03.009.
Mariadassou, Mahendra; Pellay, François-Xavier (August 2014). "Identification of amino acids in mitochondrially encoded proteins that correlate with lifespan". Experimental Gerontology. 56: 53–58. ISSN 1873-6815. PMID 24657631. doi:10.1016/j.exger.2014.03.009.
Kitazoe, Yasuhiro; Kishino, Hirohisa; Hasegawa, Masami; Nakajima, Noriaki; L. Thorne, Jeffrey; Tanaka, Masashi (2008). "Adaptive threonine increase in transmembrane regions of mitochondrial proteins in higher primates.". PLoS One. 3 (10): e3343. PMID 18836526. doi:10.1371/journal.pone.0003343.
Kitazoe, Yasuhiro; Kishino, Hirohisa; Hasegawa, Masami; Nakajima, Atsushi; Matsui, Nick; Lane, Masashi; Tanaka, Masashi (2011). "Stability of mitochondrial membrane proteins in terrestrial vertebrates predicts aerobic capacity and longevity.". Genome Biol Evol. 3: 1233–1244. PMID 21824868. doi:10.1093/gbe/evr079.
Moosmann, Bernd; Behl, Christian (2008). "Mitochondrially encoded cysteine predicts animal lifespan.". Aging Cell. 7 (1): 32–46. PMID 18028257. doi:10.1111/j.1474-9726.2007.00349.x.
Carlos Aledo, Juan; Li, Yang; Pedro de Magalhães, João; Ruíz-Camacho, Manuel; Antonio Pérez-Claros, Juan (2011). "Mitochondrially encoded methionine is inversely related to longevity in mammals.". Aging Cell. 10 (2): 198–207. PMID 21108730. doi:10.1111/j.1474-9726.2010.00657.x.
Rest, Joshua S.; Ast, Jennifer C.; Austin, Christopher C.; Waddell, Peter J.; Tibbetts, Elizabeth A.; Hay, Jennifer M.; Mindell, David P. (November 2003). "Molecular systematics of primary reptilian lineages and the tuatara mitochondrial genome". Molecular Phylogenetics and Evolution. 29 (2): 289–297. ISSN 1055-7903. PMID 13678684.
"Tuatara (Sphenodon punctatus) longevity, ageing, and life history". genomics.senescence.info. Retrieved 2017-07-27.
Clark, Mike A.; Shay, Jerry W. (1982-02-18). "Mitochondrial transformation of mammalian cells". Nature. 295 (5850): 605–607. doi:10.1038/295605a0.
Spees, Jeffrey L.; Olson, Scott D.; Whitney, Mandolin J.; Prockop, Darwin J. (2006-01-31). "Mitochondrial transfer between cells can rescue aerobic respiration". Proceedings of the National Academy of Sciences of the United States of America. 103 (5): 1283–1288. ISSN 0027-8424. PMC 1345715 . PMID 16432190. doi:10.1073/pnas.0510511103.
Hayakawa, Kazuhide; Esposito, Elga; Wang, Xiaohua; Terasaki, Yasukazu; Liu, Yi; Xing, Changhong; Ji, Xunming; Lo, Eng H. "Transfer of mitochondria from astrocytes to neurons after stroke". Nature. 535 (7613): 551–555. PMC 4968589 . PMID 27466127. doi:10.1038/nature18928.
Marlein, Christopher R.; Zaitseva, Lyubov; Piddock, Rachel E.; Robinson, Stephen; Edwards, Dylan; Shafat, Manar S.; Zhou, Zhigang; Lawes, Matthew; Bowles, Kristian M. (2017-07-21). "NADPH oxidase-2 derived superoxide drives mitochondrial transfer from bone marrow stromal cells to leukemic blasts". Blood. ISSN 1528-0020. PMID 28733324. doi:10.1182/blood-2017-03-772939.
Berridge, Michael V.; Neuzil, Jiri (2017-04-13). "The mobility of mitochondria: intercellular trafficking in health and disease". Clinical and Experimental Pharmacology & Physiology. ISSN 1440-1681. PMID 28409855. doi:10.1111/1440-1681.12764.
Plotnikov, E. Y.; Khryapenkova, T. G.; Vasileva, A. K.; Marey, M. V.; Galkina, S. I.; Isaev, N. K.; Sheval, E. V.; Polyakov, V. Y.; Sukhikh, G. T. (September 2008). "Cell-to-cell cross-talk between mesenchymal stem cells and cardiomyocytes in co-culture". Journal of Cellular and Molecular Medicine. 12 (5A): 1622–1631. ISSN 1582-1838. PMC 3918078 . PMID 18088382. doi:10.1111/j.1582-4934.2007.00205.x.
Toren, Dmitri; Barzilay, Thomer; Tacutu, Robi; Lehmann, Gilad; Muradian, Khachik K.; Fraifeld, Vadim E. (2016-01-04). "MitoAge: a database for comparative analysis of mitochondrial DNA, with a special focus on animal longevity". Nucleic Acids Research. 44 (D1): D1262–1265. ISSN 1362-4962. PMC 4702847 . PMID 26590258. doi:10.1093/nar/gkv1187.
Comments